Interrogating infrastructure starts simply, slowly. It is found in the openings in plain sight, in the re-conceptualization of our inherited systems as much as speculative proposals. After all, industrial ecology is embedded in even the most monolithic and ‘surficial’ of modern systems [0,1]. The existing interstate, for example, relies on labor, materials, capital costs, installation techniques, containerized transfer mechanisms, and legislative will; it is a mixed ‘eco-system’ of maintenance resources and operational relays that lurk behind familiar forms of automotive transport [2].
Over the past decades, a number of landscape urbanist provocations began unpacking its territories and flows, often, one material at a time: Pierre Belanger’s “Synthetic Surface” examined North American asphalt, contextualizing its climatically-driven development and on-going, organizational effects. CLUI’s “Trans-Alaska Pipeline” [3] and Kelly Doran’s “Tar Sands” both explored petroleum dependence, excavating the extended supply-chains, sited details, and longer-term trajectories of peak supply [4]. Other infrastructural components— jersey barriers, FHA standards, and the environmental costs of concrete—have been so thoroughly historicized, quantified, and fetishized in design culture that it’s hard to cite just a single source of inspiration.
The following road salt mappings extend this critical, excavative agenda. In the void between integrated roadway materials and individuated automotive consumption, these graphics trace out the largely state-supported systems that transform our highways, bi-way and city streets from seasonably passable, climatically contingent networks to eminently open, logistic lines. In advance of dilution and dispersion across sidewalks and gritty rows, millions of stockpiled tons, clearance crew hours, and budget bylines make emptiness and access possible. Thus, beginning with road salt usage in New York and the Northeast Corridor, this portrait proceeds through a nested series of quantitative geographies, uncovering the energy, envelopes, agents, trips, trade, territories, mechanisms and symbiotic scenarios of salt procurement and distribution.
Please join me in mapping the industrial ecologies of roadway melt, the openings of erasure, erosion, and emptiness.
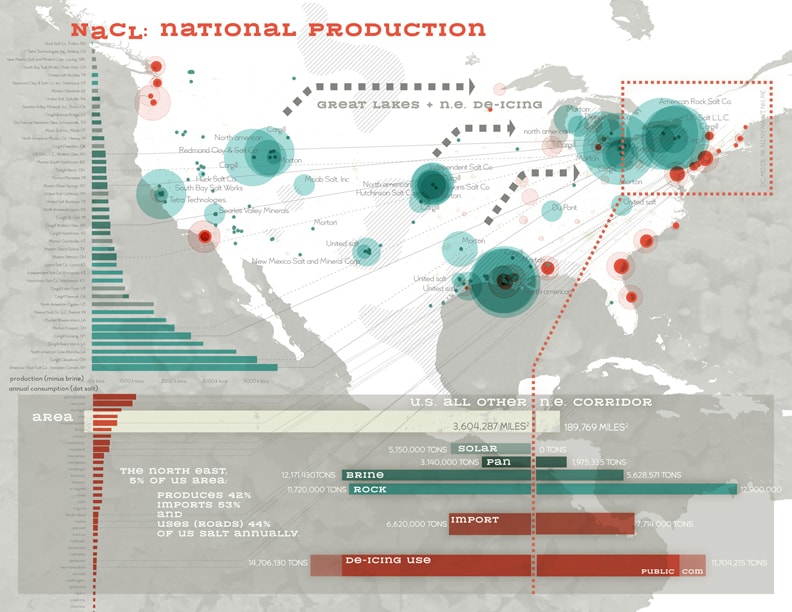
Figure 1 NATIONAL PRODUCTION: Regional resources and intensive application along the North East Corridor. Image © Meg Studer
NaCl: National Production
Today, between 52-53% of national salt consumption is devoted to seasonal roadway clearance [5]. The Northeast metropolitan corridor (unsurprisingly) consumes a disproportionate amount of this de-icing supply. Density of development, temperate jet-stream and liminal lake effect collude for climatic impact; Snowbanks sabotage J.I.T. supply chains. Blizzards bury business hours. Winter road closures cost the economy as much as $10 billion per day [6]. The Rockies and arid west may depend on snowpack for water storage and ski season, but, along the Atlantic, logistic, commerce and culture cannot wait for frozen, flaky water.
Thus, 11.7 million tons of road salt were applied here in 2008 [7]. Convenient and cheap, 42% of national stocks lie scattered between Baltimore, Boston and upstate New York, with regional resources and ease of access driving costs down to $55-65 per ton [8]. Mines beneath the Great Lakes and the Maritime Provinces serve as supplemental sources in extreme seasons. Although occasionally cities amend salt with alternate de-icers and adhesive brines, like calcium chloride and beet molasses, salt’s economic availability guarantees market hegemony.
Yet, just because salt itself is an inexpensive mineral melter, doesn’t mean that de-icing regimes are cheap. Rock salt and brine constitute, on average, 25% of de-icing budgets [9]. The larger costs of keeping roads open in the winter derive from the extensive dispersal and distribution mechanisms engaged: depot stocking, extended overtime, scrapers, spreaders, loaders, brine machines, tankers, fuel, stevedores, diesel melters, and so on… (not to mention chemical and environmental effects).
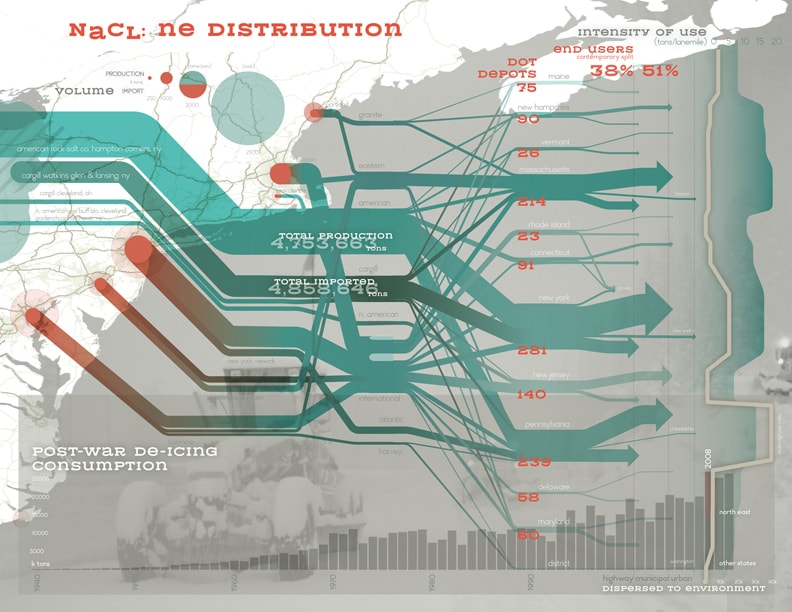
Figure 2 NE DISTRIBUTION: Between the Appalachians and the Atlantic, post-war salting has evolved into a consolidated, relatively inelastic, if geographically complex, market and municipal utility. Image © Meg Studer
NaCl: North-East Distribution
Road salt application has evolved in tandem with several modern circulation systems. The Victorians, for example, called the process salt-watering. The requisite brine was abundantly available, thanks to new mining techniques—coal prospecting—and disused Enlightenment bathing reservoirs [10]. At first, salt was applied to roads with the aim of dampening dust and stabilizing soil [11]. In 1877, HD Pearsall, CE, observed an additional salutary effect, remarking that “frost is much less liable to injure the road . . . as it rarely happens in England that the frost is severe enough to freeze salt water at all, especially at some inches under the surface” [12].
In the 1930s, engineers in Connecticut and Michigan modified this surface application [13,14,15]. By mixing salts directly into roadbed foundations, they kept the sodden, glacial clays and extreme free-thaw cycles of North American from generating radical road upheaval. In 1941, New Hampshire adopted the use of industrial spreaders for dry surface salting and gritting. Following the Eisenhower interstate build-out, other states crafted policies of their own. By the mid-50’s, New York and several other urban areas had shifted to using salt alone for its invisible, immediate maintenance demands [16].
Today, the Northeast’s consolidated yet complex salt distribution system covers nearly 90,000 miles of highway. Eight major corporations supply salt to over 1200 state DOT depots and countless municipal co-operative buyers. In Pennsylvania alone, over 1400 separate township depots require restocking each year [17,18]. With over 90% of all road salt purchases either by or through state procurement offices, salt has become a seasonal utility.
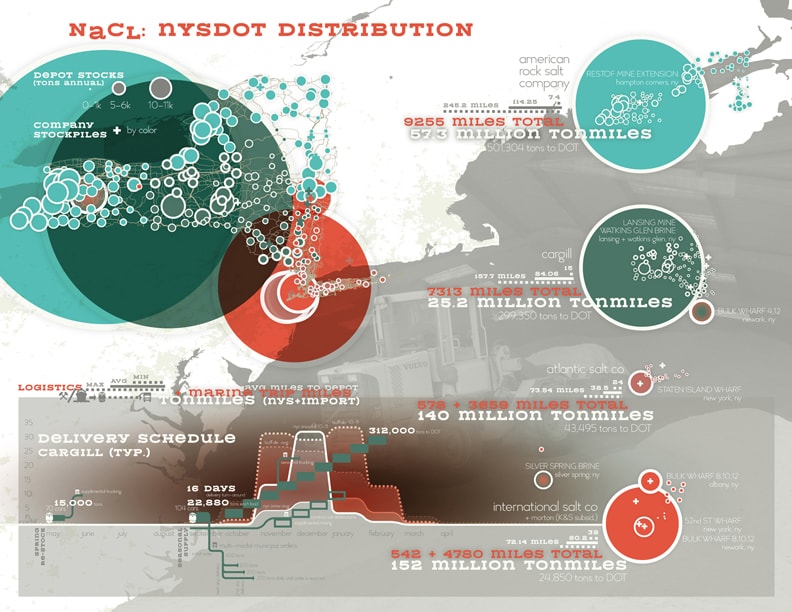
Figure 3 NYSDOT DISTRIBUTION: Corporate supply chains, depot constellations, and delivery choreography in New York State exemplify the dynamic feedback of salt for and within logistics networks. Image © Meg Studer
NaCl: NYSDOT Distribution
Salt underpins, but is also enabled by today’s vast, consolidated transport chains. New York State, the nation’s third largest salt producer and home to the nation’s third largest port, offers an interesting, if slightly amplified illustration of the logistical links between fleet ownership, inclement delivery, and the dynamics of salt sourcing. Here, resources are split between domestic production—lateral veins beneath the Finger Lakes—and international imports. Upstate, American Rock Salt, and Cargill operate mines and brine plants, as well as their own multimodal and hopper rail fleets. Cargill, Atlantic, and International Salt Co (with K+S and Morton) import vast loads of rock salt from S. America and the Caribbean to their docks in Newark, Staten Island, and Albany [19,20,21]. While Atlantic relies on chartered crafts, Cargill, and International own their own shipping lines.
Here, fleet control, computer tracking, and berth ownership have enabled the industry to stockpile supplies at distribution nodes and within circulation spaces. For example, Cargill (NY/NJ) runs over 200 hoppers in New York (out of ~1,300) and two berths at Newark’s bulk facilities. This includes 70-120 odd car trains for initial seasonal stocking. Their control of row/ sidetracks and multimodal hubs enables storage at secondary sites and selective deployment of mobile stocks [22,23]. Given that annual state consumption exceeds DOT storage capacity by somewhere between 30 to 50%, this internally distributed system enables quicker, shorter shipping legs and accommodates exceptionally snowy seasons.
In fact, New York State salt contracts are written with this distribution system in mind. Municipal customers are told that their deliveries, no matter the season, will arrive daily in staggered amounts of 600 tons, then 200 tons every day until order are fulfilled. This banks on reasonably close hopper loads, with lower daily volumes and longer lead times for larger orders [24]. It also allows townships to order salt for a week-long storm, even if they don’t have the full storage: they just need to use a bit more than those later, daily deliveries. While other states, like Connecticut, have tried to neutralize transportation entanglements by requiring full stockpiles by the first of November, in New York the salt distribution system acts as its own dynamic storage [25]
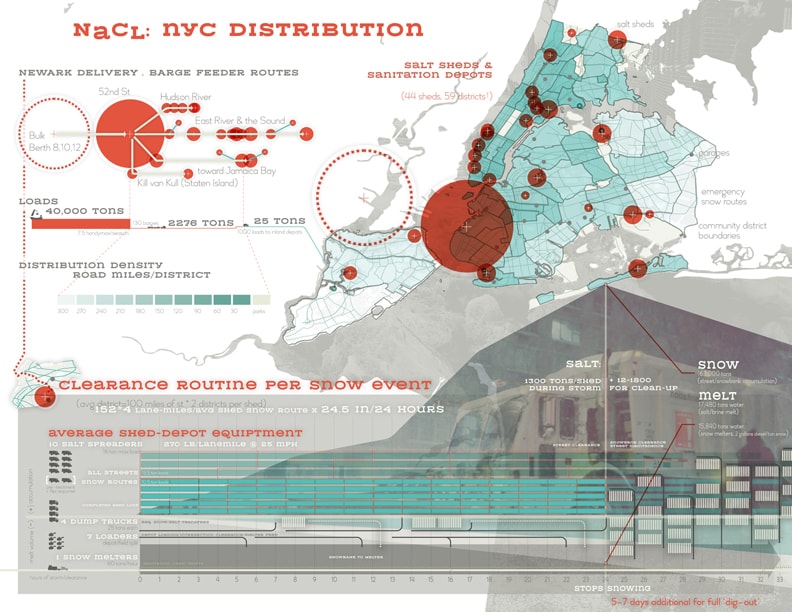
Figure 4 NYC DISTRIBUTION: Behind NYC’s ‘grid’ of easily accessible depots, the distribution of salt requires extensive overtime labor, thousands of miles of driving (and fuel), and even short-term, independent equipment contracts. Image © Meg Studer
NaCl: NYC Distribution
The port cities of New York, Boston, Philadelphia and Baltimore all fight snow with imported salt, waterborne local distribution networks, and extended sanitation shifts. For example, New York City’s annual allowance — roughly 300 thousand tons of Chilean rock salt—is supplied by International Salt Co. through the port of Newark [26]. From there, International’s feeder barges transport salt to major depots in Brooklyn, Albany, and up the Sound to New Haven [27]. In transit between (and pulling from) these stocks, International is able to deliver to roughly half of New York’s salt sheds (about 20 sit directly on or adjacent to the water, despite EPA clean-water concerns). The city sanitation department uses its 282 loaders and 149 ‘cut-down’ trucks to transfer supplies to the remaining 16 inland domes and storage piles [28,29].
In a storm event, the average depot has a little over 200 miles of road to cover and clear. Even with concentrated attention to emergency routes (152 miles with 2-4 lane clearance) it takes 5 plow-spreaders almost 3 hours to loop the emergency routes once, distributing approximately 82.08 short tons of salt per loop. Covering all the streets takes 3 salt spreaders a full 6 hours and 5 salt re-fills. An average driver clears around 450 miles, plowing just over 9,000 tons of snow in a single 12 hr shift. When a storm dumps 223,000 tons of snow per depot in a single day (January storms, 2011), complete clearance can require almost 7 days of continual salting, vehicular clearance, and mechanical melting [30,31,32]. Each year the city also contracts “equipment with operators” to supplement and speed up its own snow removal [33].
Imagine the energy, the efforts, the agents, and the imports required for a snow season expanded and intensified from Halloween blizzards to April emergencies.
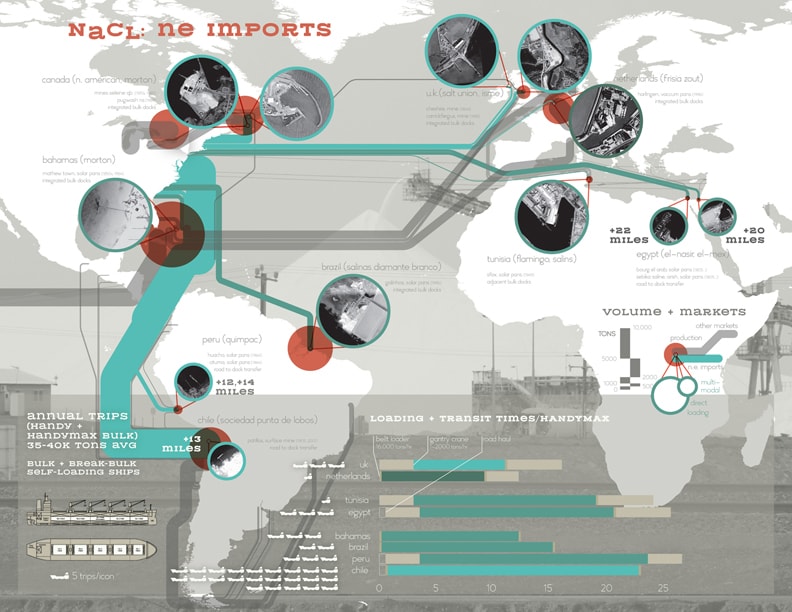
Figure 5 NE Imports: Cheap salt, imported from coastal mines and port-side pans, is largely available due to the post-war standardization and mechanization of bulk shipping. Image © Meg Studer
NaCl:NE Imports
From Baltimore to Boston, over 4.5 million tons of salt arrives annually from the Caribbean, S. America, Europe, and N. Africa [34]. The efficiencies of water transport over rail and road—lower costs, less congestion, larger loads and better fuel efficiency—have been critical for more than just NYC’s barge feeder alliance. It has captured 51% of the Northeast’s de-icing market.
The instruments of modern bulk loading—mechanized belts and massive gantry cranes—enable between 2-16,000 tons/hour to be loaded into 40,000 ton cargo holds, operating with a scale and speed lacking in land transfer [35,36,37]. Often built into today’s standardized handysize and handimax vessels, these industrial loading mechanisms make any deep river or mid-sized berth into an ideal drop or pick-up point for multimodal cargo. To a certain extent, the rise of industrialized shipping has drawn production towards the sea as well, keeping the traditional and time-intensive production of salt in solar pans and evaporative pools economically viable (in Egypt, Bahamas, Brazil, etc.).
Industrial room-and-pillar salt mining, where possible, has also evolved to profit from such water-borne transport. Like the Detroit works beneath the Great Lakes, or the Gulf-Coast domes of Louisiana, most of the Northeast’s Canadian and European imports come from mining operations adjacent to or directly under-seas. From mines at ISME (Ireland), Union (UK), Morton and N. American (CA), belt loaders snake directly from production shafts to shipping berths and customized canals. While Union’s site dates from Roman and Victorian eras, the majority of the works supplying the Northeast (and all those with internal docking facilities) have been developed in the wake of post-war shipping standardization [38]. One can say that, as a driver for today’s salt market, the need for open roads has only been matched by the supply advantages of the open seas.
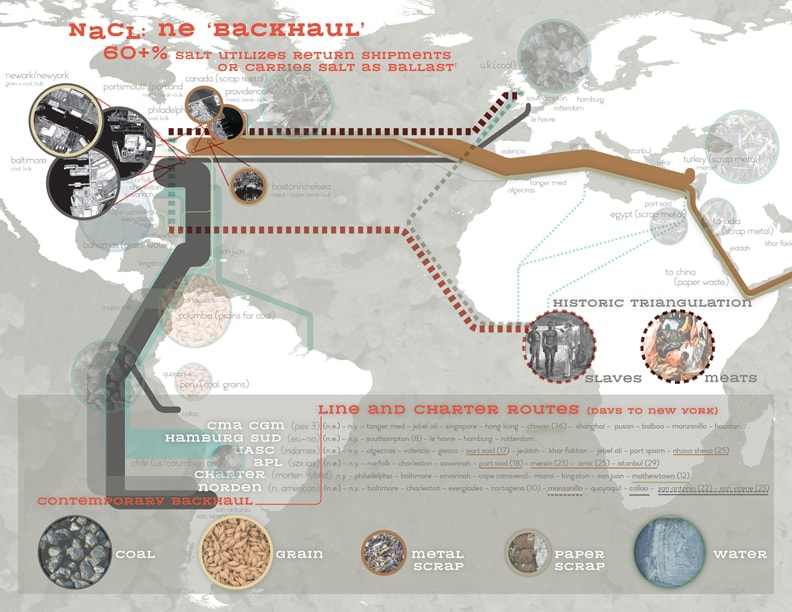
Figure 6 NE Backhaul: The industries and alliances subsidized by salt. Image © Meg Studer
NaCl: NE Backhaul
Salt imports to the Northeast are supported by more than just the technical ease of shipping: most trading partners/nations have trade agreements with the U.S.; more importantly, almost all receive ‘return’ goods from the East Coast’s major free trade zones [39,40].
Norden, the shipper hired by Empressa for International Salt Co, provides an interesting example. Norden’s ships run salt from Societas Punta de Lobos in Chile through the Panama Canal and north to Boston. They then turn south again, making further deliveries at Newark, Philadelphia, and Baltimore. As they unload salt, handi-max cargo holds are then re-filled with grains bound for Columbia and hard coal destined for South America. In Columbia, Norden ‘tops up’ with more coal, which is finally delivered in southern Chile, for electric generation [41]. Thus, one can speak of a trade triangle, in which salt is, in fact, the ‘backhaul’ good (that is, a second, separate cargo carried in the later leg(s) of a journey to split or reduce overall fuel costs). The Norden triangle capitalizes on salt’s inelastic demand to offset shipping costs for more lucrative wheat ($200-400/metric ton) and coal ($100-150/metric ton) [42]. In doing so, the Northeast’s appetite for clear streets becomes entangled in Chilean energy politics, subsidizing the expansion of coal-thermal power plants.
The New England trade routes partner salt with other goods. During the colonial era, the British triangulated Boston-bound salt with its production and use; Liverpool sent slaves to the Caribbean pans, salt to Boston, and, finally, imported the Colonists’ preserved cod [43]. Today, Boston’s Mediterranean salt imports constitute the ‘backhaul’ for bulk scrap headed to Eurasia and beyond: steel bound for Turkey and India, paper and cardboard bound for Chinese recycling. Thus as Boston’s largest import, salt also subsidizes Boston’s two largest exports [44]. Although less dramatic than coal politics or slave swapping, these break-bulk routes indicate just how embedded salt is in the mundane circulation of consumer packaging and re-purposed industrial resources.
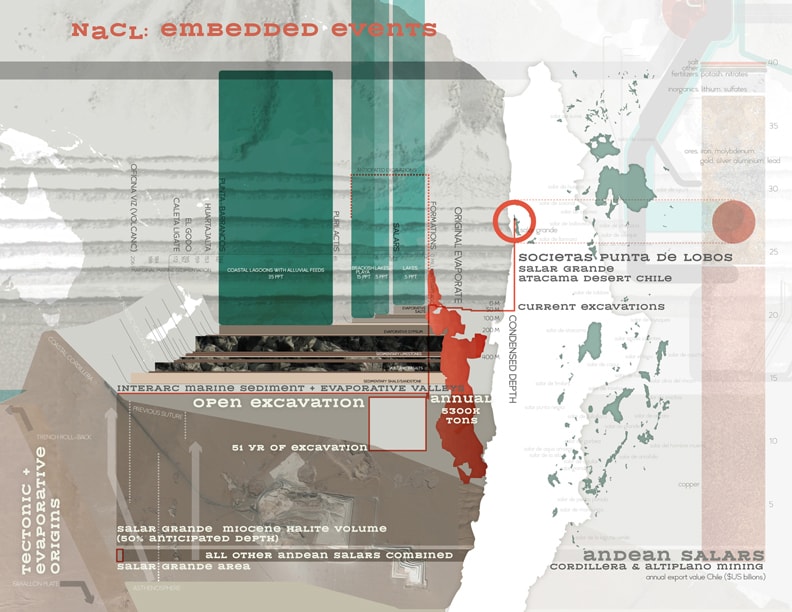
Figure 7 EMBEDDED EVENTS: Along with global goods, cumulative eons sit behind de-icing salt delivery and adjacent, immanent sourcing streams. Image © Meg Studer
NaCl: Embedded Events and Alternate Arrays
Along with hidden transit times and subsidized cargo contracts, typical Northeast road salt engages eons. Solar evaporation—used in Egypt, Tunisia, Peru and the Bahamas—requires up to five years to produce and prep a salt harvest. New York City’s salt, mined by Societas Punta de Lobos (SPL) in Chile, ranges from 10-23 million years old [45]. Excavated from the top 60-120m Salar Grande in the Atacama Desert, these salts are merely the ‘upper crust’ of an enduring arid climate. Beneath the pure sodium chloride halites lie another 150 million years and 150m of evaporation. Northern Chile’s salt is layered with nitrates, gypsum, lithium, and copper. Providing more than just salt, the salars are anticipated to become intense sites of future, ‘digital’ competition since they are currently the largest producers (42%) of refined lithium on the planet [46].
Even older, salt from upstate NY hails from nearly 350 million years ago. The lateral veins stretched beneath the Finger Lakes to Ohio are remnants of the receding shores of Rheic and Panthalassic Oceans. Considered from the vantage point of the marginally melted blocks of a New York City blizzard, the journey of salt condenses and collapses together no less than:
- 23 million years of evaporation: 24 days of shipping transit time: 1 day of tug/barge/truck loading/transfer time: and 12 hours min. as dispersed during 1 DSNY spreader application shift
- 40 k tons of hard coal as the alternate cargo during Norden hauling: 40 k tons of surrounding salt during backhaul
- 1 handimax ship @ 42.3 million ton-miles: 1 barge/tug @ 6828 ton-miles: 1 loader: 1 spreader @ 5850 ton-miles/shift and 20.2 billion BTU or 3452 barrels of oils for ocean transit; 3.078 billion BTU or 530 barrels of oil per quarried handimax load; .75 barrels of oil per spreader shift; 24 barrels of oil per shift of mechanical-snow melter use… [47]
It’s quite an impressive effort, quite an energy sink to subsidize speed, negate climate, and enable emptiness, even at the small scale of a community district or single storm. Barring the likelihood that collectively, commercially we’d embrace the frozen stasis, this stark accounting invites the question of how we might engage novel salt strategies and scales in the immediate, anticipated winters of the Northeast. Be it sited tactics (recuperating urban melt lots or appropriating fracturing brine), alternate ecological uptake (by migrating, brackish marshes, alternate aquacultures and sodic fodder crops), or recombining logistics arrays (from Columbian coal and Bolivian lithium to re-occupying the side-rails and stockpiles of New York), let us end with this open invitation to projection; what are the alternate couplings, ‘recycling’ operations, and internal arrangements we might glean and hybridize from these salt systems and sources? How does road salt’s industrial ecology, its extended, operational footprint, alter how we consider automotive infrastructure and its alternate futures?
Meg Studer is a designer, researcher, and historian with a focus on the administrative tools of territorial construction, from popular projections to embedded, operational materials. Aside salt logistics, she has forthcoming articles on environmental accounting and climate control in antebellum Boston and a show on the aerial, global (and dystopian) cartographic imagination cultivated between WWII and Apollo-8. Meg holds MAs in history and theory from the Architectural Association and Columbia University, as well as an MLA from the University of Pennsylvania. She is currently a MA candidate in Interactive Telecommunications at New York University and leads Siteations studio.
References
[0] Industrial ecology is roughly the study of material and energy flows through industrial systems, as modeled with the tools of engineering, economics, sociology, toxicology and the natural sciences. Its’ approach endeavors to analyze and understand the emergent behavior of complex, integrated human/natural systems. See Brad Allenby, “The ontologies of industrial ecology,” Progress in Industrial Ecology 3 (1/2): 28–40 and The International Society for Industrial Ecology, accessed February 17, 2013, http://www.is4ie.org/
[1] Alex Wall, “Programming the Urban Surface” in James Corner, ed. Recovering Landscape (New York: Princeton Architectural Press, 1999) 246.
[2] Pierre Belanger, “Synthetic Surfaces” in Charles Waldheim, ed. The Landscape Urbanism Reader (New York: Princeton Architectural Press, 2006) 239-295.
[3] Kelly Doran, “Tar Sands” in John Knechtel, ed. Fuel, Alphabet City no. 13 (Cambridge, MA: MIT Press, 2009) 278-307.
[4] CLUI, “Trans-Alaska Pipeline” in Rania Ghosn, ed. Landscapes of Energy, New Geographies 02 (Cambridge, MA: Harvard U Press, 2009) 75-82.
[5] Dennis Kostick, “Table 3 Salt Produced In The United States, By Type And Product Form,” “Table 6 Distribution Of Domestic And Imported Salt By Producers In The United States, By End Use And Type” in “Salt Mineral Yearbook 2008,” (adjusted production + use volumes), USGS Minerals Information, accessed October 29, 2011. http://minerals.usgs.gov/minerals/pubs/commodity/salt/index.html#myb.
[6] R. Adams, Houston, L. and, Weiher, R., “The Value of Snow and Snow Information Services,” NOAA’s National Operational Hydrological Remote Sensing Center, accessed October 29, 2011. http://www.nohrsc.noaa.gov/technology/pdf/NOAAs_National_Snow_Analyses.pdf
[7] “Salt Mineral Yearbook 2008” (total U.S. usage compared with State volumes, note 7)
[8] Sources and Prices averaged from State DOT and municipal co-op procurement contracts (2008 adj. numbers), accessed October 29, 2011.
-
-
-
Massachusetts: MA VEH40 (updated as 72, 91), Access: search ‘VEH40’ at https://www.ebidsourcing.com/
-
-
-
Pennsylvania: PA municipal Legacy Contract No 6810-02 (CN00030381), Access: search # at http://www.emarketplace.state.pa.us/ Pa 10-5050,16-2610, 3076, 4050, 4990, 5327, 5791, 6943
-
-
-
New York: NYS PC61323, PC61940-42, PC62613-4, PC63267-71. NYS OGS Delivery Schedule. Access: http://www.ogs.state.ny.us/purchase/spg/awards/01800DS00.HTM
-
Rhode Island: RI BID7322916, Access: search # http://www.purchasing.ri.gov (more recent contract # 7449026)
-
[9] This estimate is derived from PA’s state snow budget in comparison with its’ salt costs; For example, PA on average has spent $46 million annually on salt, but has a total snow removal budget of $196 million. “PA DOT Winter Operations Guide,” accessed October 29, 2011. ftp://ftp.dot.state.pa.us/public/PubsForms/Publications/PUB%20660.pdf
[11] Arthur Blanchard, “Dust Prevention by the Use of Palliatives.” The American City v 8. (Buttenheim Corp: New York, 1913) 293-297. (U Michigan Digitization), accessed October 29, 2011. http://books.google.com/books?id=R6ZLAAAAMAAJ&dq=road+salt+water&source=gbs_navlinks_s.
[12] Frank W. Grierson, “The Supply of Sea-Water to London.” American Architect and Architecture v 51, n 1051. (February 15, 1896) 74-78. (U Virginia Digitization), accessed October 29, 2011. http://books.google.com/books?id=C4lMAAAAYAAJ&dq=road+salt+water&source=gbs_navlinks_s.
[13] Cheshire, Laura. “Have Snow Shovel, Will Travel,” National Snow and Ice Data Center, accessed October 29, 2011. http://nsidc.org/snow/shovel.html
[14] Looker, et al. “Use of Sodium Chloride in Road Stabilization,” Transportation Research Board, accessed October 29, 2011. Order/abstract at http://pubsindex.trb.org/view/1939/C/122009
[15] Tiney et. al. “Progress Report of Treatment of Icy Pavements,” Transportation Research Board, accessed October 29, 2011. Order/abstract at http://pubsindex.trb.org/view/1933/C/110789
[16] Blackburn, et al. “Development of Anti-Icing Technology,” NRC Strategic Highway Research Program, accessed October 29, 2011. www.trb.org/publications/shrp/SHRP-H-385.pdf
[17] State Highway extents: “Functional System Length – 2008 Miles by Ownership” (Table HM-50) from “Highway Statistics: 2008,” Federal Highway Administration, accessed October 29, 2011. http://www.fhwa.dot.gov/policyinformation/statistics/2008/hm50.cfm
[18] State DOT and municipal co-op procurement contracts (2008 adj. Numbers), see endnote 7. PA specific DOT/Depot numbers from “Legacy Contract No. 6810-02 Sodium Chloride (CN00030381),” accessed October 29, 2011. www.dgsweb.state.pa.us/costarsreg/docs/sodiumchloride09-10.pdf
[19] NYS production and import volumes: Dennis Kostick, “Table 2 U.S. Salt Companies By Production Capacity, Location, And Type In 2008,” “Table 12 U.S. Imports Of Salt, By Customs District” in “Salt Mineral Yearbook 2008.”
[20] “Principal Ports of the United States, 2009 update,” (Salt/bulk facilities, ownership/rental/use), Navigation Data Center, US Army Corps of Engineers, accessed October 29, 2011. http://www.ndc.iwr.usace.army.mil/data/datappor.htm
[23] Car count from “The official Railway Equipment Register: Private Car Owners (April 2010),” accessed October 29, 2011. Reposted by www.progressiverailroading.com/pr/graphics/pr0810c.pdf
[24] Specific delivery schedule interpolated from NYS Salt Contract Award terms, “Award Document-Contract Award Notification: Group 01800-Road Salt” (p7), accessed October 29, 2011. http://www.ogs.state.ny.us/purchase/spg/awards/0180022287CAN.HTM
[25] Connecticut delivery terms: CT 10PSX0159 Contract Document, “Exhibit A-10.Delivery” (p3 of exhibit A), accessed October 29, 2011. Search # at http://www.biznet.ct.gov/.
[26] For general urban import information search International Salt Co (ISCO) in state contracts.
For NYC specifically see “Global Market: “Estados Unidos” Societas Punta de Lobos, accessed October 29, 2011. http://www.spl.cl/english/international/index.html.
For NYC usage volumes (salt + brine) see “DSNY: Annual Report, 2009,” NYC.gov-Sanitation Publications, (Equipment/salt use/crew shifts/ melter speeds p 6, 15), accessed October 29, 2011. http://www.nyc.gov/html/records/html/govpub/sanit1.shtml
[27] See CT contract, footnote 16 for required stockpiles. See also “Bulk Stockpiles and Salt Packaging Facilities.” International Salt, accessed October 29, 2011. http://www.internationalsalt.com/logistics.html
[28] NYC depot/sheds locations as determined from annual budget requests and garage locations:
[30] NYC GIS: “community districts” (area), “snow emergency routes,” “street center lines.” NYC Open Data, accessed October 29, 2011. http://nycopendata.socrata.com/browse?limitTo=maps
[31] Sanitation shifts, equipment capacity from DSNY Annual Report (footnote 17, reference 3). Calculation of distribution speeds and melt speeds based on best practices of driving 30 mph and Reinosdotter, et. al. “Road Salt Influence on Pollutant Releases from Melting Urban Snow.” Water Qual. Research. Canada, 2007 · Volume 42, No. 3, 153-161 Accessed 10.29.2011. http://www.cawq.ca/cgi-bin/journal/abstract.cgi?language=english&pk_article=352.
[32] Lane-miles/street mileage from “How Smooth are New York City’s Streets,” Fund for the City of New York (2001 report), c http://venus.fcny.org/cmgp/streets/pages/reports.htm
[33] “Agreement To Hired Equipment With Operators For Snow Removal Emergencies 2011-12,2012-13 And 2013-14 Winter Seasons” DSNY Contract Opportunity, accessed February 18, 2013. http://www.nyc.gov/html/dsny/downloads/pdf/business/82711SN00060.pdf
[34] General import information from Denis Kostick, “Table 11 U.S. Imports For Consumption Of Salt, By Country,” “Table 12 U.S. Imports Of Salt, By Customs District” in “Salt Mineral Yearbook 2008.”
Specific Trade Partnerships (Major imports only, North East only), accessed October 29, 2011:
From ownership links:
From other:
[38] Corporate site searches (from footnote 22) confirm site/plant ages. Loading/Logistics technology were determined from web/google imagery.
[39] U.S. Foreign Trade Zones: “Maine,” “Massachusetts,” “New York,” “Pennsylvania,” Import Administration, accessed October 29, 2011. http://ia.ita.doc.gov/ftzpage/letters/ftzlist-map.html
[40] Trade Agreements from “WTO & Multilateral Affairs,” “Free Trade Agreements,” and “Trade & Investment Framework Agreements,” Office of the United States Trade Representative, accessed October 29, 2011. http://www.ustr.gov/trade-agreements
[42] Price flux range was derived from posted wheat on Index Mundi and coal from the Energy Information Administration:
[43] Kennedy, Cynthia M. “The Other White Gold: Salt, Slaves, the Turks and Caicos Island, and British Colonialism.” Historian 69, no.2 (Summer 2007): 215-230.
[44] Martin Associates, Rhode Island’s Ports: Opportunities for Growth, (2011) with Consolidated data for New England from the U.S. Economic Census Data, accessed October 29, 2011. www.dem.ri.gov/bayteam/documents/riports.pdf
[47] Ton-miles and feight-type energy use from “Freight Facts and Figures” (2005), Table 5-7 and 5-7M: Fuel Consumption by Transportation Mode), Federal Highway Administration, accessed October 29, 2011. http://ops.fhwa.dot.gov/freight/freight_analysis/nat_freight_stats/docs/05factsfigures/table5_7.htm.
Infographic Sources:
Figure 01-NaCl: National Production
See Endnotes 4-8 and:
“Salt Historic Statistics,”USGS Minerals Information, accessed October 29, 2011. http://minerals.usgs.gov/ds/2005/140/
“Waterborne Commerce of the United States, 2008,” (Salt and Halite Shipments), Waterborne Commerce Statistics Center, US Army Corps of Engineers, accessed October 29, 2011. http://www.ndc.iwr.usace.army.mil//wcsc/wcsc.htm“Principal Ports of the United States, 2009 update,” (Salt/bulk facilities, ownership/rental/use), Navigation Data Center, US Army Corps of Engineers, accessed October 29, 2011.http://www.ndc.iwr.usace.army.mil/data/datappor.htm
Figure 02-NaCl: North-East Distribution
See Endnotes 7, 9-12 and:
“Salt Historic Statistics”
“Salt Commodity Summaries 1996-2010”
Figure 03-NaCl: NYSDOT Distribution
See Endnotes 7, 13-16 and:
Multiple State DOT/municipal contracts (2008 adj. Numbers), see footnote 7 for web-links: NYS PC61323 (Cargill), PC61940-42 (American, Cargil, N. American), PC62613-4 (American, N. American), PC63267-71 (American, Atlantic, Cargill, International)“Principal Ports of the United States, 2009 update”“American Rock Salt Case Study,” RMI Transport Management Solutions, accessed October 29, 2011. http://www.rmiondemand.com/customers/studies
Figure 04-NaCl: NYC Distribution
See Endnotes 17-20
Figure 05- NaCl: NE Imports
See Endnotes 21-23″Ocean Shipping Schedules,” (Typical liners/routes and turn-around times (region search)), Port of New York and Newark, accessed October 29, 2011. http://www.panynj.gov/port/ocean-shipping-schedules.cfm
Google Earth facilities location aerials (lat/long location):
N. American (Amherst, Nova Scotia and Gooderick, Ontario: 45.842555°, -63.656099° and 43.744905°, -81.719652°)
Union Salt (Cheshire, UK: 53.208665°, -2.518766°)
K&S:
Morton (Inagua, Bahamas: 21.000333°, -73.571522°)
Societas Punta de Lobos (Tarapaca Terminal, Chile: -20.804084°, -70.191258°)
Salinas Diamante Branco (Areia Branca-Rio Grande do Norte, Brazil: -4.971058°, -37.128942°)
Frisia Zout B.V. (Harlingen, Netherlands: 53.187514°, 5.425555°)
Eastern/Atlantic Salt trading partners:
ISME (Carrickfergus, Ireland: 54.727687°, -5.747926°)
El Nasr Salines Co (Sfax, Tunisia: 34.717084°, 10.747215°)
El Mex Salines Co. (Alexandia, Egypt: 31.089687°, 29.813206° and 31.096128°, 33.453041°)
Figure 06- NaCl: NE Backhaul
See Endnotes 24-28
“Ocean Shipping Schedules”
Figure 07- NaCl: Embedded Arrays and Alternates
See Endnotes 29-30
Jonathan Clarke, “Antiquity of aridity in the Chilean Atacama Desert,” Geomorphology2006.
Horacio Parent, “Oxfordian and Late Callovian Ammonite Faunas and Biostratigraphy of the Neuquen-Mendoza and Tarapaca Basins” Boletin del Instituto de Fisiografia y Geologia. 2006